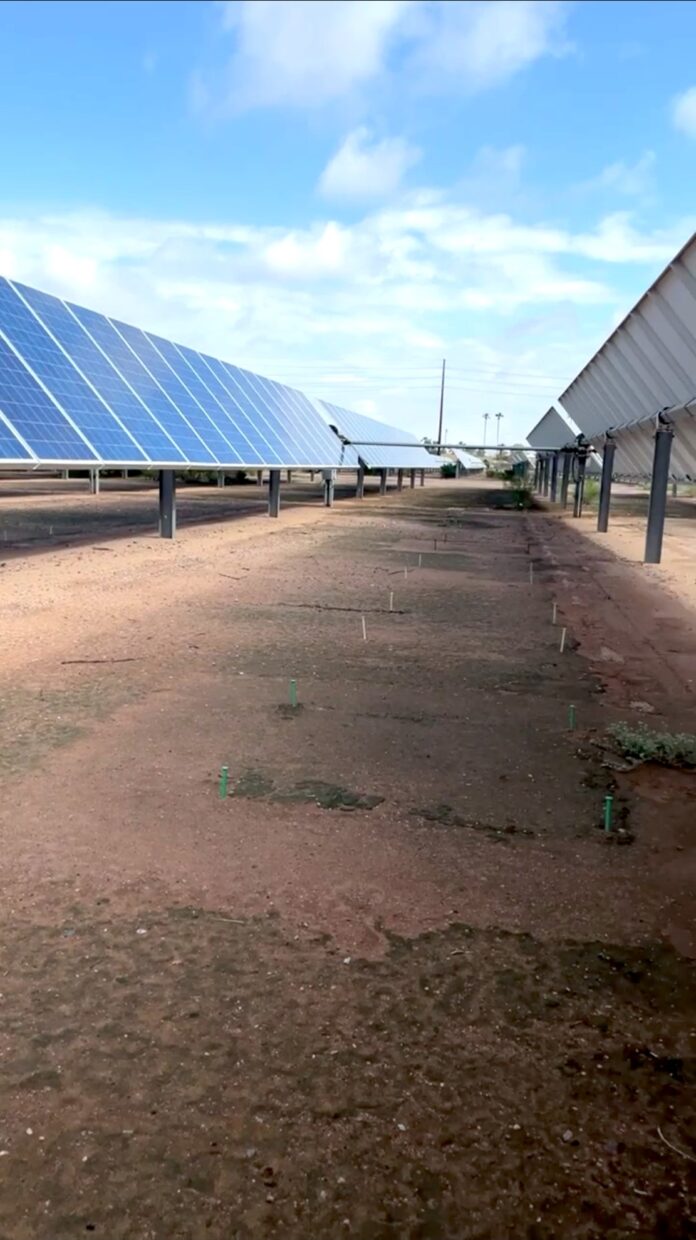
Deserts are commonly thought of as dusty places because they often are. An inherently sparse vegetation cover makes soils of arid lands more prone to wind erosion and a source of fugitive dust when winds pick up than their counterparts of greener pastures. This form of particulate atmospheric pollution is not only a common nuisance but also a pervasive public health risk to the ever-expanding population centers of arid lands.
Airborne dust loads in desert areas are significantly enhanced by certain forms of land use that include agricultural and other practices that trample the soil surface. Native desert biomes are only a very minor source of dust compared to adjacent cultivated areas under similar climate and meteorological conditions.1
One reason behind this marked difference is that native, pristine deserts harbor a natural, self-sustaining, live suit of armor: biological soil crust or biocrusts. These are communities of microorganisms that rely on photosynthesis to grow on the very top surface of desert soils, weaving soil particles together into a cohesive wind resistant cover.2 Much has been learned during the last 50 years about the ecology of this miniature, cryptic ecosystem and about the biology of the organisms that inhabit it.
Biocrusts are able to withstand extremes of desiccation, insolation and abrasion and to thrive in a sorely nutrient-poor environment. But biocrusts have an Achilles’ heel: they are brittle when dry and hence sensitive to trampling. Cattle grazing, plowing, vehicular traffic and construction all severely, even catastrophically, impact biocrusts. So much so that in vast desert expanses that now support intensive land use and were once presumably heavily colonized by biocrust, only biocrust remnants in a few untouched places remain at this point.
Scientists saw an opportunity to apply the knowledge gained towards the interventional restoration of arid soils, taking advantage of the many ecosystem services beyond erosion control that biocrust provide, including soil fertilization through carbon and nutrient drawdown from the atmosphere.3 The combined efforts of many trailblazing scientists all over the world, eventually resulted in a new kind of restoration practice that involves microbes, lichens and mosses, rather than plants. The practice involves microbial nurseries rather than tree nurseries. And yet, for all the technological advances on how to cultivate biocrust organisms in the lab or on nurseries,4 how to avoid biocrust pests,5 how to best re-introduce inoculum in the wild6 and how to promote its survival in the field,7 soil restoration through biocrusts remained an approach that required significant expertise and time investment. This limited the target footprint for application to pilot scales that reached a few hundred square meters in the best cases.
Crustivoltaics is a new approach designed to solve the scale constraints in biocrust restoration.8 The approach is based on the finding that the rows of elevated photovoltaic panels of solar farms create a milder microclimate in and over the soils that they cover, allowing much faster and more robust growth of biocrusts. Research has quantified the effect with the existing cover and biomass of biocrust under panels of a solar farm in Mesa, Arizona, USA with biocrust growth greatly exceeding that of uncovered, neighboring soils with similar climate and soil characteristics.
Other data shows that biocrusts under solar panels are no different in microorganismal composition from the natural, local biocrusts that grow without panel cover. In crustivoltaics, solar farms can serve as expansive, already existing biocrust nurseries that can produce the desired inoculants without much effort and at large scale.9 Importantly, crustivoltaics can be operated in a continuous mode, since experiments showed that harvesting biocrusts resulted in speedy recoveries as long as a small level of re-inoculation of harvested plots were carried out.
Following a rain event at a solar farm where crustivoltaics is being developed, incipient biocrusts on the soil, as well as areas that have been experimentally cleared of biocrust, and quadrats that have been re-inoculated, are obvious on the wet soils. The green-pigmented cyanobacteria migrate up to the soil surface from their refuge a few sand-grains below it10 to take advantage of optimal conditions for photosynthesis and growth (Figure 1).
A couple of climatically typical years are enough to bring biocrusts to original cover and biomass levels if the harvested areas are reinoculated to some 10% of the harvest. The remaining 90% can be used to inoculate restoration target soils outside of the solar facility in the neighboring area. Principally, biannual harvests are possible from a single source.
Crustivoltaics can increase capacity by orders of magnitude, which should suffice to reach regional scales. Researcher calculations show that the use of only the three largest solar farms in Maricopa County, Arizona as biocrust nurseries would enable a small-size business to restore the biocrust of all fallow agricultural lands in the county, which represents over 70,000 hectares (172,974 acres), in fewer than five years.
Important to this solution is that it offers additional economic and ecological incentives to solar farm operators to become active stakeholders by decreasing the load of wind-blown dust over panels, which is detrimental to energy output, and adding value to operations through production of goods (inoculants) and carbon credits (increased carbon draw-down by new biocrusts). The technology relies heavily on local solutions such as using a solar farm to restore soils in the surrounding landscapes that share soil types and climate. Local communities are thus primary stakeholders and main beneficiaries.
As a potential limitation, solar farms in geographical proximity to the restoration targets are a necessity to prevent maladaptation of the harvested biocrust to the target’s climate or soil properties. This may exclude truly remote areas from restoration. Researchers are currently working on technologies to expand the range of crustivoltaics to include restoration targets within similar climatic provinces but differing in soil composition.
Confirmatory pilot studies and initial deployment are currently being carried out or planned in central Arizona, a source of major dust pollution for the Phoenix Metro area because of long-term intensive land use, in collaboration with public state agencies and private solar operators.
References
- Finn DR, Maldonado J, de Martini F, Yu J, Penton CR, et al. 2021. Agricultural practices drive biological loads, seasonal patterns and potential pathogens in the aerobiome of a mixed-land-use dryland. Science of the Total Environment 798:149239.
- Garcia-Pichel F. 2023. The microbiology of biological soil crusts. Annual review of microbiology 77:149-71.
- Rodríguez-Caballero E, Castro AJ, Chamizo S, Quintas-Soriano C, Garcia-Llorente M, et al. 2018. Ecosystem services provided by biocrusts: From ecosystem functions to social values. Journal of Arid environments 159:45-53.
- Nelson C, Giraldo-Silva A, Garcia-Pichel F. 2020. A fog-irrigated soil substrate system unifies and optimizes cyanobacterial biocrust inoculum production. Applied and environmental microbiology 86:e00624-20.
- Bethany J, Johnson SL, Garcia-Pichel F. 2022. High impact of bacterial predation on cyanobacteria in soil biocrusts. Nature communications 13:4835.
- Faist AM, Antoninka AJ, Belnap J, Bowker MA, Duniway MC, et al. 2020. Inoculation and habitat amelioration efforts in biological soil crust recovery vary by desert and soil texture. Restoration Ecology 28:S96-S105.
- Giraldo-Silva A, Nelson C, Penfold C, Barger NN, Garcia-Pichel F. 2020. Effect of preconditioning to the soil environment on the performance of 20 cyanobacterial strains used as inoculum for biocrust restoration. Restoration Ecology 28:S187-S93.
- Heredia-Velásquez AM, Giraldo-Silva A, Nelson C, Bethany J, Kut P, et al. 2023. Dual use of solar power plants as biocrust nurseries for large-scale arid soil restoration. Nature Sustainability 6:955-64.
- Garcia-Pichel F. 2023. Using solar farms as a platform for the ecological restoration of arid soils. Nature Sustainability 6, 891–892.
- Pringault 0, Garcia-Pichel F. 2004. Hydrotaxis of cyanobacteria in desert crusts. Microbial Ecology 47:366-373.
About the Expert
Ferran Garcia-Pichel, Ph.D., is a professor of microbiology at Arizona State University. He is interested in microbial adaptations, the roles microbes play to shape their environment, including translating this knowledge gained into practical applications, particularly in ecological restoration.