New Technology and Tools Produce Effective Engineered Systems
By Corey Simonpietri
Positive results to infiltration tests create a wide range of celebratory reactions in the civil engineering community. Tiger Woods-style fist pumps and high-fiving over cubicle walls are justified because there is a lot to celebrate when a project site has the ability to infiltrate stormwater.
Taking advantage of the soil’s ability to infiltrate and treat stormwater can, at worst, benefit the design and environmental performance of the project and, at best, prove to be a panacea for applications including urban flood mitigation, meeting permit requirements for new construction, using green infrastructure to mitigate overflows from combined sewers and water quality retrofits.
Infiltration is compatible with regulatory requirements. If the local reviewing authority requires runoff reduction or reductions in sediments, phosphorus, nitrogen, or other pollutants, infiltration can provide the solution. Infiltration also meets permit requirements for peak flow rate reduction or the use of energy balance equations.
Unfortunately, infiltration testing results are often too low—less than 1 inch per hour—to use in a reliable infiltration design. That leaves engineers to face the daunting task of resolving stormwater challenges using a basket of best management practices (BMPs) that address the symptoms of development instead of the challenges of meeting quantity and quality permit requirements that are created when rainfall turns into runoff. Detention systems reduce the peak flows of stormwater discharges without addressing the volume, and water quality systems remove a percentage of some of the pollutants while discharging other, mostly untreated, pollutants. These BMPs can consume large chunks of otherwise useable land, drive up capital costs and increase long-term maintenance costs.
What if there was a way to engineer an infiltration system to increase the infiltration capacity of the soil? Just as a constructed wetland provides benefits in places where a wetland does not naturally exist, improvements to in-situ soils can be engineered—allowing for the movement of water between particles to filter pollutants and reduce or eliminate stormwater runoff from a project site.
Infiltration Basics
The first step to improve infiltration is to understand why some soils won’t infiltrate. In a publication called Soil Infiltration, the National Resource Conservation Service (NRCS) says that “soil texture, or the percentage of sand, silt, and clay in a soil, is the major inherent factor affecting infiltration.”1 The number and size of pores that affect water movement into and through the soil are influenced by the texture and structure of the soil.
Soils, particularly in areas where soil characteristics have been altered by development, tend to have multiple layers with texture and structure variations from one layer to the next. Sometimes, even soils with the right physical characteristics for infiltration suffer from one stubborn layer that refuses to cooperate. NRCS says that infiltration is often based on “how fast water can move through the most restrictive layer, such as a compacted layer, or a layer of dense clay.”2
However, the presence of clay soils or a layer of compacted or uncooperative soil in the profile doesn’t preclude the use of infiltration. The United States Environmental Protection Agency notes that low impact development (LID) practices “can be sited on clay soils if… the infiltrative capacity of the soils has not been significantly altered,” as when “compacted by construction activities or previous land uses.”3
Even though water can move through even the most difficult of soils, designing for infiltration in a predictable manner is no simple task. Reliable designs must account for both soil chemistry and compaction that may exist naturally or occur during development and post-development activities. Accounting for those factors requires an understanding of the forces that pull water through soils—gravity and capillary action.
Just as gravity pulls rainwater from the sky to the ground, so does gravity pull water from the surface down through the soil profile. Less predictable is capillary action, which is the attractive force of soils which pulls water laterally and vertically into the pore spaces between soil particles. Generally speaking, the smaller the pores, the greater the capillary forces and, therefore, the slower or lower infiltration rate. Of course, capillary action is also a function of moisture content as well as pore size, which means that it decreases as moisture content increases.
Traditional infiltration relies on gravity to pull water through the larger openings between soil particles. Sandy soils—NRCS Hydrologic Soils Group A & B4—that consist of larger particles, typically have larger voids for water to flow and are associated with better infiltration rates. Innovative engineered infiltration systems are not typically needed for sandy soils, as the traditional infiltration basins are usually adequate.
In soils made of up smaller particles, which are typical of NRCS Hydrologic Soils Group C & D found on the most infuriating of projects, gravity is less effective due to the smaller pores between soil particles. Engineered infiltration systems use capillarity to draw water laterally, making it available over a much wider footprint for gravity-based infiltration, evaporation, and transpiration.
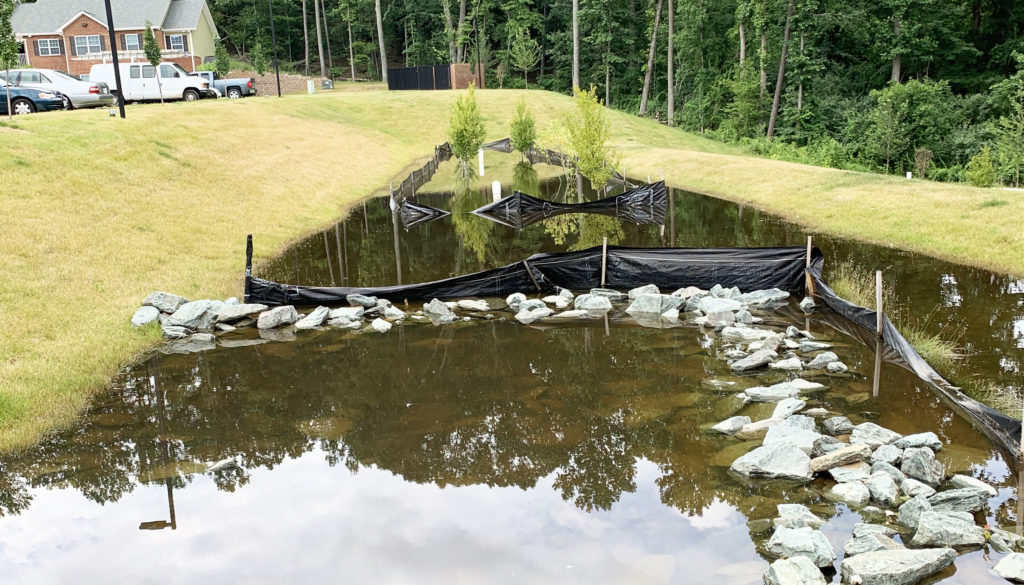
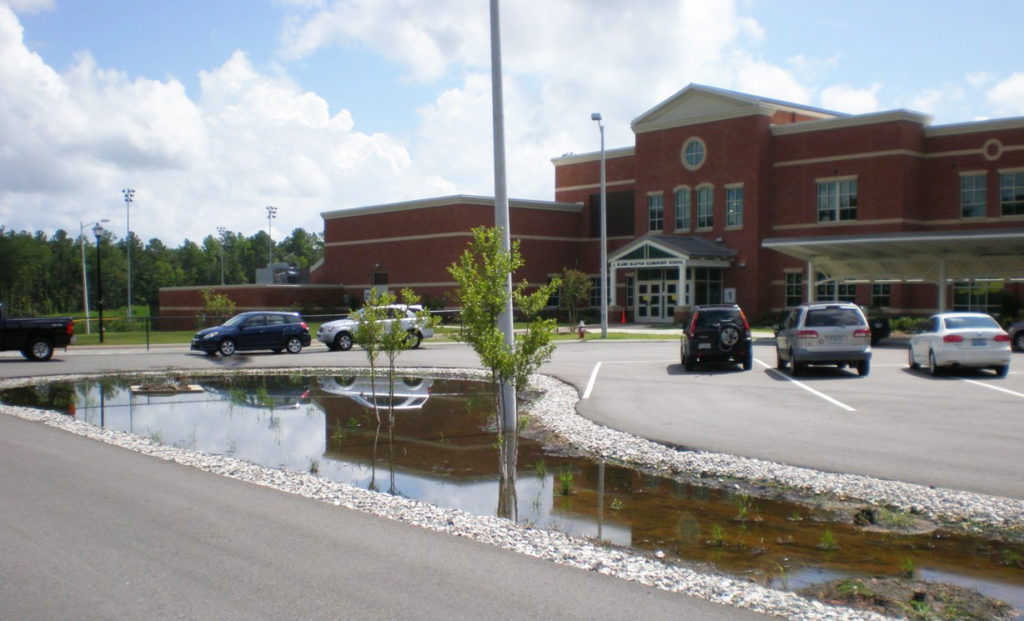
Improving Infiltration
A variety of techniques have been used to increase infiltration rates of soils. Farmers use cover crops; residential construction uses compost amended soils and urban planners use trees in green infrastructure systems. Trees and their roots may provide the best model to restore infiltration capacity to a soil. As they grow longer, tree roots penetrate soil layers to hydraulically connect multiple tiers, allowing water to pass between them more easily. Tree roots also grow wider, creating macropores—pathways for water to flow in the soil. Multiple studies have found trees to be an effective way to improve infiltration.5,6
Replicating the effectiveness of tree roots is not a simple process, and engineering a predictable outcome is challenging. Some engineers have tried using perforated pipes installed vertically or backfilled auger-drilled holes with drainage rock—all with mixed results. While some level of general improvement might be acceptable when designing retrofits, it is not adequate when designing for new construction. When designing a reliable infiltration system becomes too difficult to overcome the time and expertise challenges of developing a reliable plan, there are companies that provide the opportunity to outsource the engineering.
While engineered infiltration systems are common in the wastewater world, they are relatively new to the stormwater field. A combination of infiltration models that incorporate site specific conditions and design goals along with local design parameters such as drainage areas and time of concentration are used to develop an engineered infiltration system specific to a single project.
These systems may combine traditional and new approaches that complement each other to improve infiltration and management of stormwater. Starting with the traditional approaches and working toward the innovative, these systems and practices include:
• Mulches, ground cover and native vegetation to reduce compaction of soil.
• Amending soils with compost to improve soil health and increase infiltration.
• Hydraulically applied soil microbes that improve soil retention capacity
and general soil health.
• Underground detention systems and bioretention systems that combine underground storage reservoirs using stone or modular tanks with an elevated outlet to promote infiltration.
• Suspended pavement systems in urban areas prevent soil compaction from vehicular and pedestrian traffic to encourage infiltration, and they typically include street trees to help absorb water.
• Trees and shrubs or technology that mimics the performance of tree roots to balance moisture content across the soil profile. Some innovative systems use a vertical shaft that replicates the performance of a tree root to create flow paths between soil layers and can improve existing infiltration capacity. Research shows that areas using these systems are significantly drier than control areas in the study.7
Whoever you turn to for assistance with an engineered infiltration system, there are three important elements critical to the success of a project:
A design concept is needed for the project plans, along with a model that demonstrates the system’s ability to meet the requirements of the project to satisfy site owner and plan reviewer expectations. Construction guidance is important to ensure the system can be constructed properly, and a maintenance plan is useful to ensure the system performs as designed throughout its design life.
A performance warranty is provided for any proprietary system. This is critical as the final plan will likely be stamped by the local engineering firm.
Other Considerations
While different proprietary systems may have different components, any infiltration system can benefit from establishing good pre-treatment. From grass filter strips to plunge pools to inlet screens, there are a multitude of options that will prove beneficial over the long term.
As with traditional systems, limitations exist with engineered infiltration systems. Elements like karst and separation from the water table must be examined, but they tend to play a smaller role when capillarity dominates the design, and water tends to move more laterally than vertically. While more research is needed, the work that has been done to date shows no relevant changes to groundwater level and quality,8 even in systems where proximity of the water table would have made a traditional infiltration system violate regulatory limitations.
While the prospect of reviewing and inspecting an engineered infiltration system can be intimidating, it is important to note that the post-installation verification process is the same as any other stormwater system installed on a site. Engineered infiltration systems can be observed during and after a storm to ensure that they are drawing down as expected. If they are not, they can usually be rehabilitated more easily than traditional systems, many of which require a complete removal and replacement. Rehabilitation of an engineered systems that use vertically-installed tubes only require the installation of additional tubes.
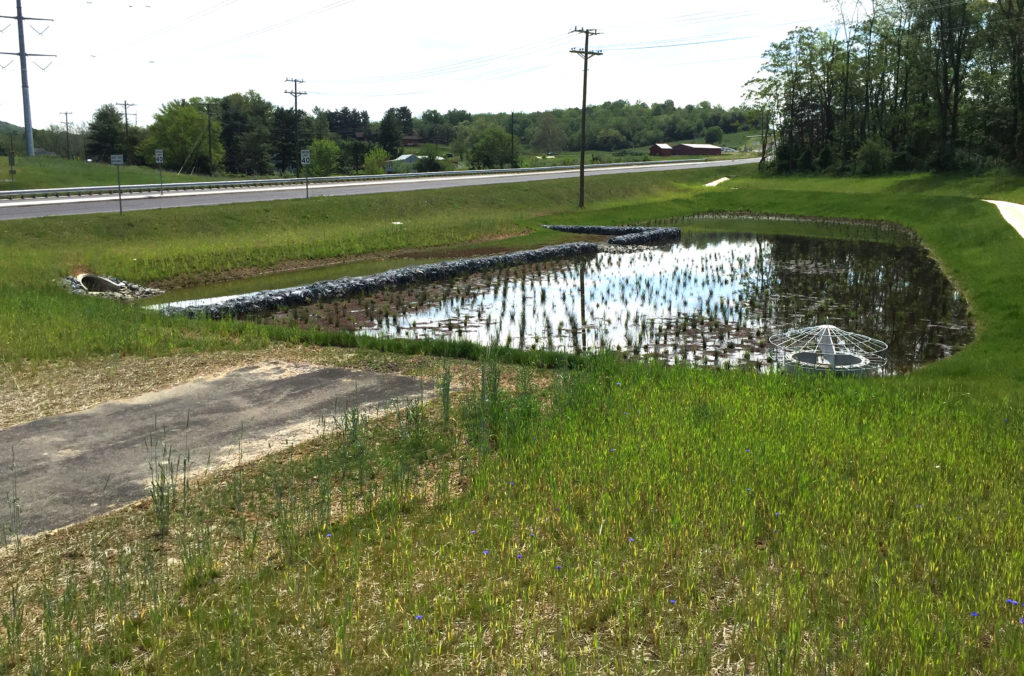
Words of Encouragement
When your next infiltration test comes back with disappointing results, explore the possibilities of restoring the infiltration capacity of the soils on the project. It is quite likely that an engineered infiltration system can greatly simplify the design, freeing up space for other elements of the project. Better yet, engineered infiltration systems frequently cost less than using stormwater BMPs to manage runoff quality and quantity.
However, the greatest benefit of infiltration is a project that sustainably reduces runoff and pollutant transport to help protect the local watershed. When totaled up, all of these results are well deserving of at least one good fist pump.
References
- Soil Infiltration: Soil Health – Guides for Educators. National Resource Conservation Services, May 2014. https://www.nrcs.usda.gov/Internet/FSE_DOCUMENTS/nrcs142p2_051576.pdf.
- Soil Infiltration: Soil Quality Kit – Guides for Educators. National Resource Conservation Services. https://www.nrcs.usda.gov/Internet/FSE_DOCUMENTS/nrcs142p2_053268.pdf.
- Soil Constraints and Low Impact Development. U.S. Environmental Protection Agency. October 2014.
- USDA NRCS National Engineering Handbook, Part 630, Chapter 7 – Hydrologic Soil Groups. NRCS, Washington DC, 2009.
- Bartens et al. Can Urban Tree Roots Improve Infiltration through Compacted Subsoils for Stormwater Management? Journal of Environmental Quality, 2008; 37 (6): 2048.
- Lunka, P. D., Lunka, P., & Patil, S. D. (2015). Impact of tree planting configuration and grazing restriction on canopy interception and soil hydrological properties: Implications for flood mitigation in silvopastoral systems. Hydrological Processes, 30(6), 945–958. https://doi.org/10.1002/hyp.10630.
- Nikolai, TA. 2017 Statistical Analysis Summary. Michigan State University. January 10th, 2018.
- Environmental Consulting and Technology, Inc. (ECT). “EGRP®Pilot Demonstration Project on Belle Isle, Detroit Michigan Technical Memorandum” February 10, 2016.
About the Expert
Corey Simonpietri is the director of Stormwater Management at ACF Environmental (now part of Ferguson Enterprises) and has worked in the geotechnical and stormwater field for 25 years. An avid paddler of low skill but ample enthusiasm, he is a champion of the environment and relishes the challenge of balancing human development with protection of natural resources.