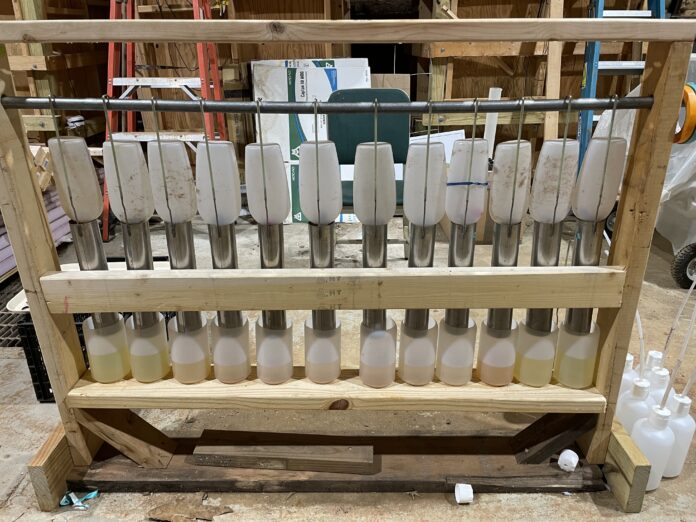
Water used to wash clothes and dishes, take baths and showers, flush toilets and mix with cleaning products makes its way from houses to wastewater treatment plants for people on public water and wastewater systems. After passing through the wastewater treatment process, the treated water is either discharged to the local waterbody or land applied for infiltration into the soil profile. At this point, wastewater system operators must also consider the erosion and sediment control practices that are in place to avoid contamination of waterways due to runoff after precipitation events.
Products that contain per- and poly-fluroalkyl substances (PFAS) or “forever chemicals” are commonly used in homes. These are synthetic chemicals that were originally synthesized in the 1930’s1 and have since found many different uses where waterproofing needs are desired. Some products that contain PFAS are Teflon™ pots and pans, waterproofing compounds, makeup and carpeting. PFAS are comprised of carbon-based compounds in which the carbon has been chemically bonded to fluorine atoms. With the high electronegativity of these carbon-fluorine bonds, a high activation energy is required to break these bonds making treatment and removal challenging.2,3
At some wastewater treatment plants, instead of discharging the treated water into a waterbody, the treated water is sprayed on a land application system (LAS). Land application systems can consist of grassed fields, forest or a combination of the two. These systems are covered under NPDES permits, and there is a requirement that no runoff should occur from the facility. However, during precipitation events, there could be runoff from the site causing soil erosion. This stormwater could carry chemicals on the surface of the LAS or attached to eroded soil particles. Around the LAS is a buffer zone to slow down and retain any soil, nutrients and other chemicals contained in the runoff. There have been some studies of buffer zones and their ability to retain and reduce nutrient levels as the water moves through the buffer zone, but there has been little to no research on the buffer zones’ ability to retain PFAS. A study is currently being conducted to answer some of these questions, but results are not currently available as samples from the project are being analyzed.
Along with the current research on buffer zone retention of PFAS, researchers at the University of Georgia are investigating the potential of using stormwater practices such as bioretention basins or modified versions to retain and remove PFAS prior to final discharge to local waterbodies. Initial studies involved the use of column studies to determine removal percentages of PFAS from a solution of six PFAS chemicals typically found in wastewater treatment plant effluent and applied to LAS. This solution consisted of the PFAS chemicals, perfluorooctanesulfonic acid (PFOS), perfluorooctanoic acid (PFOA), perfluorononanoic acid (PFNA), 6:2 fluorotelomer sulfonate (6:2 FTS), perfluorobutane sulfonic acid (PFBS) and perfluorohexane sulfonic acid (PFHxS). The objective of the column study is to determine if a combination of materials available at LASs in both North Georgia and South Georgia could be used to retain and reduce the concentrations of PFAS leaving LASs from precipitation-induced runoff.
In Georgia, the northern portion of the state is classified as Piedmont, and the soil profile is comprised of clay-based soils. The southern portion of the state is a coastal plain, and the soil profile is comprised of loamy sand soils. This study investigated the use of these two types of soil (a Pacolet Clay representing North Georgia and a Tifton Loamy Sand representing South Georgia) which could be found on the LAS site mixed with sawdust. Blends were made of soil and sawdust in ratios of 100%/0% (soil/sawdust) to 0%/100% with 20% changes in each portion of the blend. Each of the six mixtures was added to small stainless-steel columns that were 5.5 cm (2.2 inches) in diameter and 28 cm (11.0 inches) in length with screen mesh on the bottom to contain the mixture. Once the mixture was added, the columns were dropped from a height of 10 cm (4 inches) onto a surface to provide a consistent packing of the mixture. The columns were then hung from a rack and 500 mL (16.9 ounces) of liquid containing either a tracer dye or PFAS water was introduced to leach through the mixture. Collected samples were analyzed for fluorescence or PFAS concentration (Figure 1).
The analysis of PFAS removal percentages for the clay and sawdust (Figure 2) shows that as the percentage of clay decreases in the mixes, the removal percentages of PFAS also decreased. Some of this is related to the PFAS chemical in itself. The chain length of PFAS affects adsorption or desorption; shorter chains partition to water and longer chains are preferential to soil.4,5 The cation exchange capacity of the soil/sawdust mixture appears to have some affect on the removal pecentages of PFAS. As sawdust becomes the predominate portion of the mixture, the fluoresence of the collected sample, as indicated by the tracer dye values, increases. Some trees will naturally have a fluoresence due to the componds in lignin of the tree.6 The sawdust used for this experiment was collected from a local sawmill where the wood consisted of conifers as well as hardwood.
As the percentage of loamy sand decreases from 100% to 20%, there is not much change in the removal percentages of PFBS, PFHxS, PFOA and 6:2 FTS (Figure 3). PFOS and PFNA have higher removal rates in the 100/0 mixture while the percentage of removal generally decreases with increasing sawdust until the 0/100 mixture. At 0% soil, five of the chemicals increase in removal percentage. With this soil being a loamy sand, the attraction to the soil particles could be causing a lower adsorption rate and therefore a reduced removal percentage for the PFBS, PFHxS, PFOA and 6:2FTS. Clay soil typically has a negative charge, while sandy soil may have slight negative or no charge.
The research is continuing, but the potential of using on-site materials as well as materials that are collected from citizens in county/city-based debris removal can potentially be cost-effective ways to capture and retain some of the PFAS that potentially could be in precipitation-induced runoff from LAS sites where this system is used.
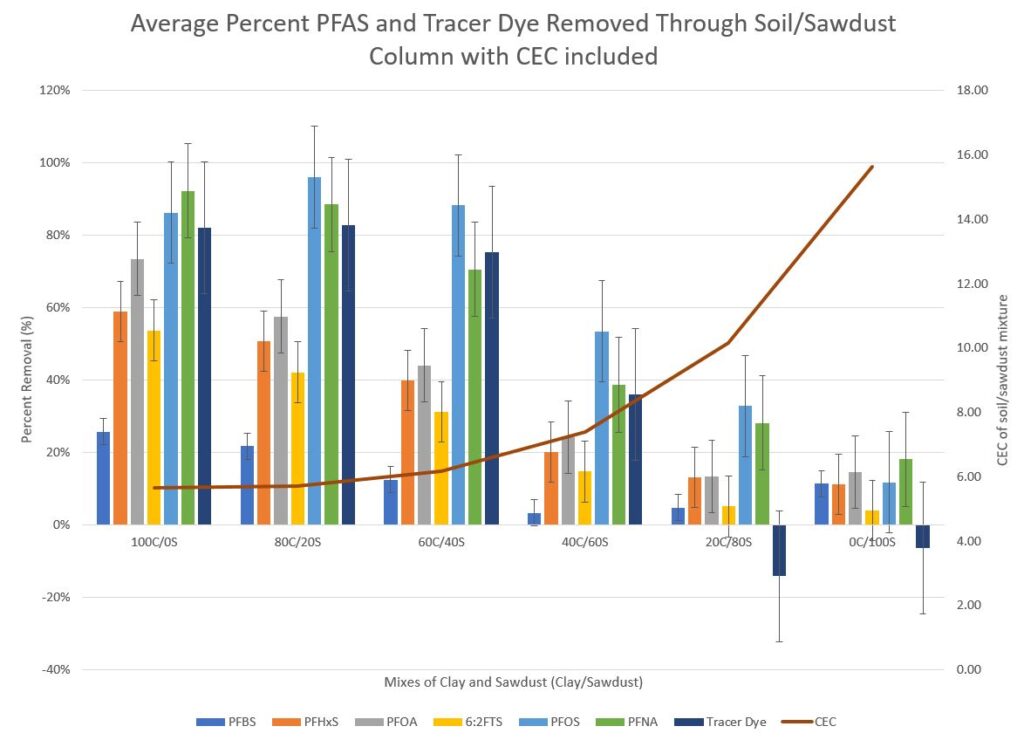
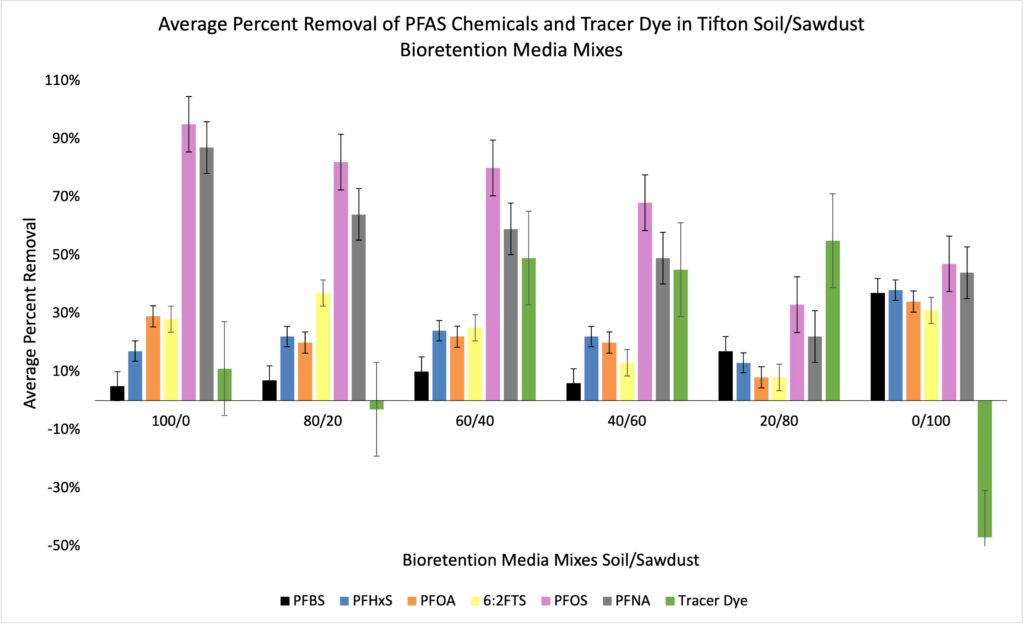
References
- Moyer M. (2021). “Forever Chemicals”: PFAS Contamination and Public Health. Penn State Law Review, 2021. https://www.pennstatelawreview.org/print-issues/forever-chemicals-pfas-contamination-and-public-health/. Last viewed 16 January 2024.
- Zhang Z, Sarkar D, Biswas J K, et al. (2021). Biodegradation of per- and polyfluoroalkyl substances (PFAS): A review. Bioresource Technology, 2021, 1–11. https://doi.org/10.1016/j.biortech.2021.126223.
- Drenning P, Volchko Y, Ahrens L, et al. Comparison of PFAS soil remediation alternatives at a civilian airport using cost-benefit analysis. Science of the Total Environment, 2023, 1-11. http://dx.doi.org/10.1016/j.scitotenv.2023.163664.
- Huang Y, Liu S, Zi J, Cheng S, et al. In Situ Insight into the Availability and Desorption Kinetics of Per- and Polyfluoroalkyl Substances in Soils with Diffusive Gradients in Thin Films. Environmental Science and Technology, 2023, 7809-7817. https://doi.org/10.1021/acs.est.2c09348.
- Seo S, Son M, Shin E, et al. Matrix-specific distribution and compositional profiles of perfluoroalkyl substances (PFASs) in multimedia environments. Journal ofHazardous Materials, 2019, 19–27. https://doi.org/10.1016/j.jhazmat.2018.10.012.
- Djikanovic D, Kalauzi A, Radotic K, et al. Deconvolution of Lignin Fluorescence Spectra: A Contribution to the Comparative Structural Studies of Lignins. Russian Journal of Physical Chemistry A, 2007, Vol. 81, No. 9,pp. 1425–1428. DOI: 10.1134/S0036024407090142.
About the Experts
Gary L. Hawkins, Ph.D., is an associate professor and water resource management extension specialist at the University of Georgia.
Bailey Williams, B.A., is a graduate student in the University of Georgia Crop and Soil Science Department.